The calibration and validation of ocean color satellites and the acquisition of
data for the development of remote sensing algorithms, requires sensor and data
accuracies of the highest order, generally better than those achieved
until now; the same data with comparable accuracies are required for the
validation of atmospheric correction algorithms and the validation of the
biogeochemical products derived from remotely sensed observations. For
SeaWiFS and other ocean color sensors, the goal is to produce water-leaving
radiances with a 5% accuracy in clear-water regions [Hooker and Esaias,
1993]. This objective requires field instruments with a calibration and
measurement accuracy that is of the order of 1%. Calibrating optical
sensors before and after a cruise rarely delivers this goal, but recent
developments of the SeaWiFS Project produced the SQM-a self-contained,
portable, and stable light with integral optical assemblies-which can be
used to check the optical stability of radiometers deployed in situ,
throughout the course of a research cruise lasting four weeks or more.
The engineering design and characteristics of the SQM are described by
[Shaw et al. 1997] and [Johnson et al. 1997], so only a brief description
is given here. The SQM is capable of monitoring the stability of radiance
and irradiance sensors to within 1% in the field. Used in conjunction with
laboratory calibration sources, the SQM can be used to transfer the
calibration to the field equipment. Three flux levels are possible, using
two lamp assemblies. The exit aperture of the SQM is large, homogeneous in
radiance, and was designed to approximate a lambertian radiator. An
internal heater provides operational stability and decreased warm-up
intervals. Temperature-controlled silicon photodiodes with colored glass
filters are mounted inside the SQM and monitor the stability of the
generated light field. The independent monitors normalize the flux of the
source, so the actual change in the responsivity of the field sensor can be
determined. A change in the responsivity of the field sensor may be
distinguished from a change in the reflectance of the front surface of the
sensor using three reference devices, or fiducials, that are designed to
mimic the reflectivity of the optical surface of the field sensor, but are
not functioning detector units. The front surface of the fiducial is
protected when not in use and is kept clean during the field experiment. A
kinematically designed D-shaped collar is used on both the optical sensors
and the fiducials to ensure the devices being tested view the same part of
the SQM aperture each time they are used.
The SQM was deployed on the AMT-3 cruise for the first time and
demonstrated the following capabilities [Hooker and Aiken, 1997]:
1. The SQM can be used to track the stability of field radiometers at less
than the 1% level in terms of the radiometric response of the sensors;
2. The SQM light field is sufficiently stable to model changes in the
radiometric detectors;
3. Based on the radiometers used during AMT-3, daily SQM measurements are
required to resolve short-term temporal changes of the radiometric
detectors; and
4. The field assessment showed that the SQM performance decayed
approximately 0.6% during the course of the 36 day deployment.
SeaOPS is composed of an above-water and in-water set of instruments
[Robins et al., 1996]. The in-water component is composed of a
downward-looking radiance sensor (OCR-200) which measures upwelling
radiance, Lu, and an upward-looking irradiance sensor (OCI-200) which
measures downwelling irradiance, Ed. The in-water instruments are mounted
on a T-shaped frame which is lowered and raised through the water column by
a winch; data are collected during the lowering and raising of the frame.
The above-water component, an OCI-200, measures the incident solar
irradiance at the ocean surface, Ed(0+). The OCI-200 and OCR-200
radiometers have seven channels, which were chosen to correspond with the
SeaWiFS instrument wavelengths and bandwidths [Hooker and Esaias, 1993].
The UOR is a measurement platform which is towed approximately 400 m
behind a ship [Aiken and Bellan, 1990]. It uses a programmable servo that
controls the attitude of a diving plane which causes the vehicle to
undulate through a preset depth range, typically 10-80 m, at tow speeds
between 10 to 12 kts. At speeds in excess of 6 kts, the servo unit is
powered by an alternator, which is driven by a propeller on the rear of the
body. During AMT-3, an OCI-200 was fitted to the top of the UOR instrument
bay to measure downwelling irradiance, Ed, and an OCR-200 was fitted to the
bottom of the instrument bay to measure upwelling radiance, Lu. The
OCI-200 and OCR-200 radiometers employed in the UOR and SeaOPS use 16-bit
analog-to-digital (A/D) converters and are capable of detecting light over
a four decade range.
SeaFALLS is composed of two subsystems: a SeaWiFS Profiling Multichannel
Radiometer (SPMR) and a SeaWiFS Multichannel Surface Reference (SMSR). The
former measures Ed and Lu
as it falls freely through the water column,
while the latter measures the incident solar irradiance just below the sea
surface, Ed(0-). The profiler receives its power and sends its data via an
umbilical cable, while the reference floats just below the surface
suspended from a square floating frame [Waters et al., 1990]. Both the
profiler and the reference can be deployed far away from the ship, so any
ship-induced disturbances to the in situ light field are minimized [Mueller
and Austin, 1995]. Since SeaFALLS can be deployed quickly by only two
people, the ship can be stopped when light conditions are optimal. SeaFALLS
is equipped with 13-channel OCI-1000 and OCR-1000 radiometers, which employ
24-bit A/D converters, and are capable of detecting light over a seven
decade range.
|
The data acquired by these means are used to validate the performance and
accuracy of algorithms for the interpretation of SeaWiFS imagery and
generate new algorithms where deficiencies are detected. Along with the
HPLC pigment measurements, the analysis points to the validation of the
Platt-Longhurst hypothesis [Platt et al., 1995; Sathyendranath et al., 1995] of bio-optical provinces
with distinct optical properties; AMT measurements show that these align
precisely with physical provinces determined by T-S relationships.
Significantly, the analysis shows that interprovince bio-optical
variability is driven by phytoplankton accessory pigment concentrations,
particularly photoprotective carotenoid (PPC) abundance, and ratio to
photosynthetic carotenoids (PSC), i.e., PPC/PSC, which is highest in
high-light equatorial zones; though this is as expected ecologically, the
ratios are province-specific rather than intensity-specific.
With the wide diversity of ecosystems encountered (two hemispheres and two
seasons are sampled over a period of 30-35 days), an AMT cruise is like
many cruises rolled into one, making it inevitable that new observations
and new discoveries will emerge on every transect. For the future, the
project will continue to pioneer new sensors and new technology. During
AMT-4, a new free-fall radiometric instrument called the Low-Cost NASA
Environmental Sampling System (LoCNESS) and a new hyperspectral radiometer
called SeaSPEC were both tested. By AMT-5, a Fast Repetition Rate
Fluorometer (FRRF) will be deployed in the UOR to provide measurements of
photosynthetic parameters in all the provinces of the Atlantic Ocean - data
which are necessary to exploit remotely sensed observations of ocean color
for estimating global primary production.
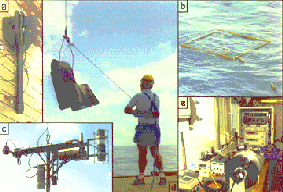
Figure 2. A collage of the core optical instruments deployed on the AMT
cruises: a) SeaFALLS profiler, b) SeaFALLS reference, c) SeaOPS, d) the
UOR, and e) the SQM.
|
References
Aiken, J., and I. Bellan, "Optical Oceanography: An Assessment of a Towed
Method." In: Light and Life in the Sea, P.J. Herring, Ed., Cambridge
University Press, 39-57, 1990.
Hooker, S.B., and W.E. Esaias, "An Overview of the SeaWiFS Project." Eos,
Trans. AGU, 74, 241-246, 1993.
Hooker, S.B., and J. Aiken, "Calibration Evaluation and Radiometric
Testing of Field Radiometers with the SeaWiFS Quality Monitor (SQM)." J.
Atmos. and Oceanic Tech., (submitted), 1997.
Joint Global Ocean Flux Study, "JGOFS Core Measurements Protocols." JGOFS
Report No. 6, Scientific Committee on Oceanic Research, 40 pp, 1991.
Johnson, B.C., P-S. Shaw, S.B. Hooker, and D. Lynch, "Radiometric and
Engineering Performance of the SeaWiFS Quality Monitor (SQM): A Portable
Light Source for Field Radiometers." J. Atmos. and Oceanic Tech.,
(submitted), 1997.
Mueller, J.L., and R.W. Austin, "Ocean Optics Protocols for SeaWiFS
Validation, Revision 1." NASA Tech. Memo. 104566, Vol. 25, S.B. Hooker,
E.R. Firestone, and J.G. Acker, Eds., NASA Goddard Space Flight Center,
Greenbelt, Maryland, 66 pp, 1995.
Platt, T., S. Sathyendranath, and A. Longhurst, "Remote Sensing of Primary Production in the Ocean: Promise and Fulfilment" Phil. Trans. R. Soc. Lond. B348, 191-202. 1995
Robins, D.B., A.J. Bale, G.F. Moore, N.W. Rees, S.B. Hooker, C.P.
Gallienne, A.G. Westbrook, E. Marañón, W.H. Spooner, and S.R. Laney,
"AMT-1 Cruise Report and Preliminary Results." NASA Tech. Memo. 104566,
Vol. 35, S.B. Hooker and E.R. Firestone, Eds., NASA Goddard Space Flight
Center, Greenbelt, Maryland, 87 pp, 1996.
Sathyendranath, S., A. Longhurst, C.M. Caverhill, T. Platt, "Regionally
and seasonally differentiated primary production in the North Atlantic."
Deep-Sea Res., 42(10), 1773-1802, 1995.
Shaw, P-S., B.C. Johnson, S.B. Hooker, and D. Lynch, "The SeaWiFS Quality
Monitor-A Portable Field Calibrator Light Source." Proc. SPIE, 2,963,
772-776, 1997.
Waters, K.J., R.C. Smith, and M.R. Lewis, "Avoiding Ship-Induced
Light-Field Perturbation in the Determination of Oceanic Optical
Properties." Oceanography, 3, 18-21, 1990.
This article appeared in the August 1997 issue of the backscatter magazine,
published by the Alliance of Marine Remote Sensing (AMRS).
|